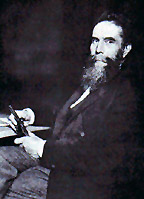
German physicist
Wilhelm Roentgen
A new form of radiation was discovered in 1895 by Wilhelm Roentgen, a German physicist. He called it X-radiation to denote its unknown nature. This mysterious radiation had the ability to pass through many materials that absorb visible light. X-rays also have the ability to knock electrons loose from atoms. Over the years these exceptional properties have made X-rays useful in many fields, such as medicine and research into the nature of the atom.
Eventually, X-rays were found to be another form of light. Light is the by-product of the constant jiggling, vibrating, hurly-burly of all matter.
Like a frisky puppy, matter cannot be still. The chair you are sitting in may look and feel motionless. But if you could see down to the atomic level you would see atoms and molecules vibrating hundreds of trillions of times a second and bumping into each other, while electrons zip around at speeds of about a million miles per hour.
When charged particles collide--or undergo sudden changes in their motion--they produce bundles of energy called photons that fly away from the scene of the accident at the speed of light. In fact they are light, or electromagnetic radiation, to use the technical term. Since electrons are the lightest known charged particle, they are most fidgety, so they are responsible for most of the photons produced in the universe.
Light can take on many forms. Radio waves, microwaves, infrared, visible, ultraviolet, X-ray and gamma radiation are all different forms of light.
The energy of the photon tells what kind of light it is. Radio waves are composed of low energy photons. Optical photons--the only photons perceived by the human eye--are a million times more energetic than the typical radio photon. The energies of X-ray photons range from hundreds to thousands of times higher than that of optical photons.
The speed of the particles when they collide or vibrate sets a limit on the energy of the photon. The speed is also a measure of temperature. (On a hot day, the particles in the air are moving faster than on a cold day.)
Very low temperatures (hundreds of degrees below zero Celsius) produce low energy radio and microwave photons, whereas cool bodies like ours (about 37 degrees Celsius) produce infrared radiation. Very high temperatures (millions of degrees Celsius) produce X-rays.
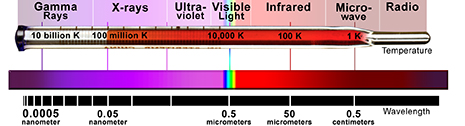
The Electromagnetic Spectrum.
The wavelength of radiation produced by an object is usually related to its temperature.
The photons themselves can also collide with electrons. If the electrons have more energy than the photons, the collision can boost the energy of the photons. In this way, photons can be changed from low-energy photons to high-energy photons. This process, called Compton scattering, is thought to be important around black holes, where matter is dense and has been heated to many millions of degrees.
The photons collected in space by X-ray telescopes reveal the hot spots in the universe--regions where particles have been energized or raised to high temperatures by gigantic explosions or intense gravitational fields.
Where do such conditions exist? In an astonishing variety of places, ranging from the vast spaces between galaxies to the bizarre, collapsed worlds of neutron stars and black holes.
Emission and Absorption Lines
When a free-ranging electron is accelerated by the electric field of a proton or charged atom (ion), the photons emitted can have a wide range of energies that depends on how fast the electrons are moving and how much they are accelerated. The distribution of photon energies due to this process is called a continuous spectrum, and can be graphed as a smooth curve.
In contrast, if the electron is in orbit around the nucleus of a neutral or charged atom (a.k.a. ion), the spectrum is a series of sharp peaks, or lines. This happens because the orbits of electrons in an atom are strictly regulated by the rules of quantum theory. These orbits, or more accurately, energy states, are separated by a specific amount of energy, just as stairs are separated by a specific height. Just as you cannot move to a position between stair steps, an electron in an atom cannot move to a position between energy states. The atoms for each element, such as oxygen, carbons, etc., have their own unique sets of energy states.
Normally the electrons in atoms are in the lowest energy state, at the bottom of the stairs. But if the atom has been excited by a collision with a free electron, another atom, or a photon, the lowest energy level will be unoccupied. One of the orbiting electrons will quickly jump down to this level, releasing energy in the form of a photon of a specific energy. These photons give rise to an emission line in the spectrum. A hot gas composed of many atoms will give off a spectrum composed of many emission lines due to the various elements that are present in the gas.
The opposite process can also occur. If a stream of photons encounters a gas, those photons whose energy corresponds to energy levels in an atom a step will be absorbed by the atom. This process gives rise to a series of absorption lines in the gas.
Careful studies of the energies of the photons emitted or absorbed by an atom of a particular element give a blueprint for the energy states of that atom. Knowing this blueprint, or energy spectrum, astronomers can look for it in the radiation from stars and gas, and determine the amount of each element present. In this way, astronomers have determined that stars are mostly made of hydrogen, with a mixture of helium and traces of heavier elements such as carbon, nitrogen, oxygen, and so on.
X-ray fluorescence from atoms occurs when a high energy particle or X-ray knocks an electron free from the innermost energy level of an atom, creating an unstable atom. An electron from an outer energy level immediately jumps into a lower energy state, with the emission of an X-ray with a distinct energy specific to the atom. Around black holes this happens when high-energy X-rays produced by hot gas very near the black hole collide with iron atoms in cooler gas and dust in the vicinity.
Another process for producing emission lines is charge-exchange. A charged ion, for example, a carbon or oxygen ion, collides with a neutral atom or molecule and captures one of its electrons. A photon is emitted as the captured electron drops to a lower energy state. This process is called "charge exchange" because an electron is exchanged between a neutral atom and an ion. After such collisions, X-rays are emitted as the captured electrons move into tighter orbits. Charge exchange is especially important for comets, where ions in the solar wind collide with neutral atoms in the cometary atmospheres.
Synchrotron Radiation
But this is not the whole story. X-ray photons can also be created under different conditions. When physicists were operating the first particle accelerators, they discovered that electrons can produce photons without colliding at all. This was possible because the magnetic field in the accelerators was causing the electrons to move in large spirals around magnetic field lines of force. This process is called synchrotron radiation.
In the cosmos particles such as electrons can be accelerated to high energies– near the speed of light– by electric and magnetic fields. These high-energy particles can produce synchrotron photons with wavelengths ranging from radio up through X-ray and gamma-ray energies.
Synchrotron radiation from cosmic sources has a distinctive spectrum, or
distribution of photons with energy. The radiation falls off with energy less
rapidly than does the spectrum of radiation from a hot gas. When synchrotron
radiation is observed in supernova remnants, cosmic jets, or other sources, it
reveals information about the high-energy electrons and magnetic fields that are
present.
[More Information]