Trying to Understand the Milky Way's Black Hole
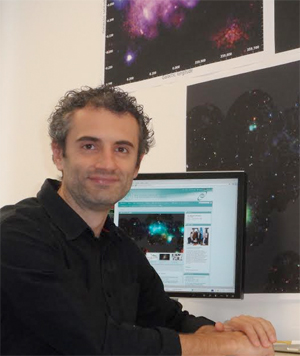
Gabriele Ponti
Dr. Gabriele Ponti is the Marie Sklodowska-Curie EU Research Fellow at the Max Planck Institute for Extraterrestrial Physics in Germany. Prior to that, he was a post-doctoral fellow at the University of Southampton in the UK, after spending a year at Cambridge University’s Institute of Astronomy. Dr. Ponti earned his Ph.D. from Bologna University in Italy before moving on to the Laboratories Astro-Particule et Cosmologie in Paris. His doctoral thesis topic was studying relativistic effects in bright active galactic nuclei and he has been interested in this area since then.
As a boy, I read about the existence of black holes for the first time. I still remember the fascination of trying to grasp the physical concepts behind one of the weirdest manifestations of nature.
Black holes produce an enormous gravitational pull, as a consequence of being extremely compact: a significant amount of mass concentrated in a very small volume.
Indeed, black holes have mass and size, in the same way as, for example, a human body, a car, the Earth and the Sun have mass and size. These two quantities are essential in determining the gravitational pull at the surface of any body. For example, to escape the gravitational pull at the surface of the Earth, a rocket has to move away with a speed of at least 11.2 kilometers per second (25,000 miles per hour). If the mass of the Sun would be compressed inside the radius of the Earth, the escape velocity would be much higher, about 6,500 kilometers per second (14,500,000 miles per hour). Of course, if even more mass (thousands of times the mass of the Sun) would be concentrated inside the Earth’s radius, the escape velocity would be higher than the speed of light. Since nothing can travel faster than the speed of light, nothing could leave the surface of such an object. This massive and compact body would, therefore, be a black hole.
The presence of a black hole has a deep influence on the surroundings, deforming the space-time around it, and anything passing nearby. I still remember that, in order to describe the peculiar effects experienced by a body falling into a black hole, the book I read as a youth used to take the human body as an example. Luckily, my interest in black holes was deep enough to overcome the horror of imagining how a human body would be deformed while falling into a black hole. Even more luckily, black holes are distant enough that no human body has ever fallen (or will ever fall) into one of them.
The research described in the Chandra press release started after fall 2012, when a team led by Stefan Gillessen showed evidence for an extended dusty object, dubbed G2, approaching at high speed the supermassive black hole at the center of our Galaxy. Unlike the human bodies of physics books, Gillessen and collaborators calculated that G2 would not directly fall into the black hole, but would rather come very close to it in its orbit. They estimated this close approach would happen around fall 2013/spring 2014, at a distance of only 20 light hours from the hole (about 150 times the Earth-Sun distance). G2 would be close enough to experience macroscopic effects, such as stretching of its dusty extended component.
Several teams made a significant effort to predict the details of how the event would unfold and to determine whether the G2 passage would have any observable effect on the hole and its surroundings. However, the results critically depended on the unknown internal structure of the G2 object, and on the interaction with the hot plasma outside the hole (of which we still have an incomplete knowledge), thus several possible scenarios were proposed. The scientific community was divided among those expecting fireworks and those predicting absolutely no additional effect, apart from a tidal stretching of the extended component of G2.
At the time of the discovery of G2, we were already studying the present and past activity of the supermassive black hole, and monitoring its X-ray emission. Inspired by this discovery, and to better constrain the physical processes at work in the interaction between G2 and the hole, we decided to increase the frequency of the X-ray monitoring. The data that we collected before and during the close approach of G2 did not show any peculiar behavior. However, our last datasets, obtained in fall 2014 (about 6 months after the close approach), showed five relatively strong X-ray flares, during a period when less than one was expected, based on the black hole’s past activity. This aroused our curiosity. Was this behavior significantly different from the commonly observed activity around the black hole? Could it be that we were witnessing the first signs of activity induced by the passage of G2? Or were the X-ray properties changing without any direct connection with G2?
To answer these questions we re-analyzed all the X-ray observations of Chandra and XMM-Newton pointed at the black hole over the past fifteen years. This allowed us to carefully characterize the X-ray flaring activity of the region immediately around the hole. We discovered that, at the end of 2014, the hole had indeed experienced a significant change of its X-ray activity, manifested by the larger number of flares observed. From monitoring in the infrared band, we now know that the main body of G2 survived the close encounter. However, a small part of the extended dusty envelope has probably been captured by the black hole, and it is expected to ultimately fall into it on a time-scale of years. This additional supply of material could have caused the observed variation of X-ray activity. In fact, theoretical investigations based on simulations have shown that the emission in the X-ray band is very sensitive to small changes in the feeding of the black hole, suggesting that this might indeed be the first band to show signs of enhanced activity induced by the passage of G2. On the other hand, we know that G1, an object very similar to G2, approached the hole at a similar distance around spring 2001. However, there has been no particular evidence for anything unusual happening as a result of G1’s passage, even though, back then, the X-ray monitoring was much sparser.
A possible alternative is that the X-ray activity from the surroundings of the hole constantly exhibits small variations. Flares might occur in clusters, instead of being uniformly distributed in time. This means that the probability of observing a flare would change over time. According to this hypothesis, the connection with G2 would be just coincidental, and we had been able to detect the variations around the time of the close passage of G2, simply because of the increased frequency of our X-ray monitoring. In agreement with this interpretation, we observe that other black holes with similar accretion rates (but black hole masses millions of times smaller) do show long-term modulation in their flaring properties.
At present, we don’t know whether the observed variation has anything to do with G2 or not and we are eager to know what the new data collected in 2015 will tell us.
Please note this is a moderated blog. No pornography, spam, profanity or discriminatory remarks are allowed. No personal attacks are allowed. Users should stay on topic to keep it relevant for the readers.
Read the privacy statement